Effects of global climate change on European marine biodiversity
This article discusses global warming and the range of effects on marine systems. The effects may be related to changing water temperatures, changing water circulation or changing habitat; as a consequence of these changes, altered pathways within biogeochemical cycles and food webs are detected as well. In the first case, the biological responses and impacts result from the physical effects.[1]
Even without human-induced climate change, the biodiversity and biogeography of species is continuously changing (seasonal and yearly changes). Consequently, long term monitoring is necessary in order to evaluate these processes. The marine systems however may become more dynamic and variable due to climate change.[1]
Coastal ecosystems of the enclosed seas such as the Baltic, the Mediterranean and the Black Sea are most threatened by climate change. These seas have only small and primarily east-west orientated movement corridors, which may restrict northward displacement of organisms in these areas.[2]
Contents
- 1 Effects on primary production
- 2 Effects on the recruitment process
- 3 Effects on the biogeography
- 4 Effects on the phenological relationships and community structure
- 5 Effects on the establishment of invasive species
- 6 Effects on biogeochemical cycles
- 7 Effects at the level of physiological responses to temperature rise
- 8 See also
- 9 References
Effects on primary production
Higher temperatures and enhanced stratification could affect the productivity of phytoplankton. A number of models predict an increase in global primary production of between 1% and 8% by 2050, when compared to pre-industrial times.[3]
Because phytoplankton is an important basis of the marine food web, any change in the timing, abundance or species composition of the phytoplankton will have an effect on the whole food web.[1]
Effects on the recruitment process
The population dynamics of a lot of marine vertebrates and fish are driven by recruitment processes. The recruitment of cold temperate species is often synchronized with seasonal production cycles of phytoplankton. Increasing sea water temperatures may advance the timing of reproduction of these fish species; this may result in a mismatch with their food source (phytoplankton) (match/mismatch hypothesis). A change in recruitment success will lead to shifts in species composition.[1]
Example: cod recruitment in the North Sea
The Atlantic cod (Gadus morhua) recruitment in the North Sea, in the past 40 years, was influenced by changes at the base of the food web (bottom-up-control), induced by the rise of temperature. Cod recruitment decreased from the mid-1980s, coincident with unfavorable changes in the plankton ecosystem.[4]
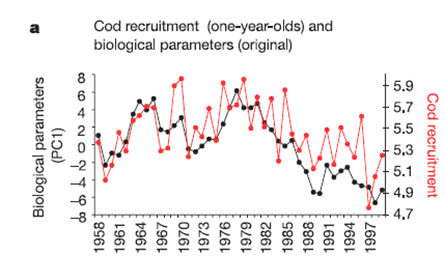
Effects on the biogeography
The species movement in a warming area is towards the poles in general. Since global warming accelerated in the late 1980s, pole ward advances of southern species and retreats of northern species have been recorded in zooplankton, fish and benthic species.[5] .[6]
The species distribution is not always northwards. For example when the stock of the main prey of the harp seals in the Barents Sea collapsed, these seals migrated southwards along the coast of Norway and into the North Sea in search of food.[1]
Example: Study of barnacles in the Celtic-Biscay shelf
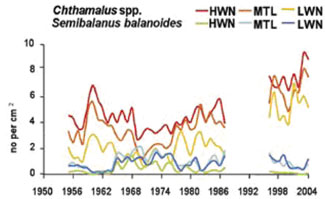
The Celtic-Biscay shelf was liable to warming in the 1930; in the 1960 there was a switch back to colder. Changes in species assemblages were described on rocky shores, using barnacles as a sensitive indicator of wider changes in marine life.[7] [6] These showed switches between warm water barnacles in the 1950s (Chthamalus spp.) to greater dominance by the cold water barnacle Semibalanus balanoides in the 1960s and 1970s. On rocky shores warm water barnacles now exceed the levels found in the 1950s.[1]
There are also examples from the North Sea and the Baltic Sea.
Effects on the phenological relationships and community structure
The response to climate changes differs between the species, inducing a decoupling of phenological relationships (relative timing of life cycle events). The decoupling may affect the community structure and food webs by altering the interactions between a species and its competitors, mutualists, predators, prey or pathogens. For example, in the case of seabirds, chick diet composition during development is likely to be an important mechanistic link between climate variability and the observed decline in seabird populations.[8]
A study in Kongsfjorden (79 °N) concluded that changing temperatures have a direct and very immediate influence on the species composition of plankton as well as on the biodiversity. The response of benthic organisms to rising temperature is slower and less drastic compared with planktonic organisms. Planktonic organisms may be a better indicator of global warming-driven changes than the benthic organisms. However benthic fauna is a good indicator of slow changes, especially when observed over a long period of time.[9]
Effects on the establishment of invasive species
The establishment of non-indigenous species can be accelerated by rapid warming. For example, the recent warming has accelerated the adaptation of the Pacific oyster (Crassostrea gigas) on the local circumstances in the Netherlands and the UK.[10]
Effects on biogeochemical cycles
In the past 200 years the oceans have absorbed approximately half of the CO2 produced by fossil fuel burning and cement production. Calculations indicate that this uptake of CO2 has led to a reduction of the pH of surface seawater, known as Ocean acidification. The reduction amounts to 0.1 units; this is an equivalent to a 30% increase in the concentration of hydrogen ions. If the CO2 emissions from human activities rise on current trends then the average pH of the oceans could fall by 0.5 units by the year 2100.
This fall in pH may have a huge impact on marine organisms, in particular to calcifying organisms such as most mollusks, corals, echinoderms, foraminifera and calcareous algae. Seawater has to be supersaturated with calcium and carbonate ions to ensure that once the biogenic calcareous structures are formed, it does not dissolve. Lower pH reduces the carbonate saturation of the seawater, making calcification harder.
There is also a difference in vulnerability between the groups of organisms: corals and a group of mollusks (pteropods) precipitate aragonite; coccolithophores and foraminifers produce the less soluble calcite. Furthermore differs the function (e.g. metabolic or structural function) from the carbonate between the different groups and this is coupled to the sensitivity for acidification. It is likely as CO2 levels increases, changes of species composition will occur because of the different responses of the species. An altered species composition may have a huge effect on the global carbon cycle.[11]
Effects at the level of physiological responses to temperature rise
Temperature rise for the oceans as a whole is likely to be about 1 to 2 °C, in the next decades. Exceptions are semi enclosed marine lagoons and shallow bays that may mirror the atmospheric temperature rise as well. Additional, most aquatic sandy-shore animals are adapted to rapid changes in temperature and they seldom experience temperatures close to their upper tolerance limits. Further, sandy-beach animals are capable of burrowing and of escaping below the sand if conditions at the surface become hostile. [12] This is in contrast to sessile organisms such as corals and mangroves that are unable to keep up with the higher temperature level.
See also
- Natural variability and change in coastal ecosystems
- Effects of climate change on the Mediterranean
- Effects of climate change on the North Atlantic benthos
- Effect of Climate Change in the Baltic Sea Area
- Climate change leads to Arctic food shortages
References
- ↑ 1.0 1.1 1.2 1.3 1.4 1.5 Phillipart C.J.M. (ed.) (2007). Impacts of climate change on the European marine and coastal environment: ecosystems approach. European Science Foundation, Marine Board: Strasbourg, France. 82pp.
- ↑ Nicholls, R.J.; Klein,R.J.T. (2005). Climate change and coastal management on Europe's coast, in: Vermaat, J.E. et al. (Ed.) (2005). Managing European coasts: past, present and future. pp. 199-226.
- ↑ Sarmiento, J.L.; Slater, R.; Barber, R.; Bopp, L.; Doney, S.C.; Hirst, A.C., Kleypas, J.; Matear, R.; Mikolajewicz, U.; Monfray, P.; Soldatov, V.; Spall, S.A.; Stouffer, R. (2004). Response of ocean ecosystems to climate warming. Glob Biogeoch Cycles 18,3. cit. in: Philippart, C.J.M. (Ed.). (2007). Impacts of climate change on the European marine and coastal environment: ecosystems approach. ESF Marine Board Position Paper. European Science Foundation, Marine Board: Strasbourg, France. 82 pp.
- ↑ Beaugrand, G.; Brander, K.M.; Lindley, J.A.; Souissi, S.; Reid, P.C. (2003). Plankton effect on cod recruitment in the North Sea.Nature (Lond.) 426(6967): 661-664.
- ↑ Brander, K.; Blom, G.; Borges, M.F.; Erzini, K.; Henderson, G.; MacKenszie, B.R.; Mendes, H.; Ribeiro, J.; Santos, A.M.P.; Toresen, T. (2003). Changes in fish distribution in the eastern North Atlantic: Are we seeing a coherent response to changing temperature? ICES Mar Sci Symp 219: 261-270. cit. in: Philippart, C.J.M. (Ed.). (2007). Impacts of climate change on the European marine and coastal environment: ecosystems approach. ESF Marine Board Position Paper. European Science Foundation, Marine Board: Strasbourg, France. 82 pp.
- ↑ 6.0 6.1 Southward, A.J.; Langmead, O.; Hardman-Mountford, N.J.; Aiken, J.; Boalch, G.T.; Dando, P.R.; Genner, M.J.; Joint, I.; Kendall, M; Halliday, N.C.; Harris, R.P.; Leaper, R.; Mieszkowska, N.; Pingree, R.D.; Richardson, A.J.; Sims, D.W.; Smith, T.; Walne, A.W.; Hawkins, S.J. (2005). Long term oceanographic and ecological research in the western English Channel. Adv Mar Biol 47: 1-105 cit. in: Philippart, C.J.M. (Ed.). (2007). Impacts of climate change on the European marine and coastal environment: ecosystems approach. ESF Marine Board Position Paper. European Science Foundation, Marine Board: Strasbourg, France. 82 pp. Cite error: Invalid
<ref>
tag; name "Southward2005" defined multiple times with different content - ↑ 7.0 7.1 Southward, A.J.; Hawkins, S.J.; Burrowa, M.T. (1995). Seventy years` observations of changes in distribution and abundance of zooplankton and intertidal organisms in the western English Channel in relation to rising sea temperature. J Therm Biol 20: 127-155. cit. in: Philippart, C.J.M. (Ed.). (2007). Impacts of climate change on the European marine and coastal environment: ecosystems approach. ESF Marine Board Position Paper. European Science Foundation, Marine Board: Strasbourg, France. 82 pp. Cite error: Invalid
<ref>
tag; name "Southward1995" defined multiple times with different content - ↑ Kitaysky, A.S.; Kitaiskaia, E.V.; Piatt, J.F.; Wingfield, J.C. (2005). A mechanistic link between chick diet and decline in seabirds? Proc Biol Sci Proc R Soc B 273:445-450. cit. in: Phillipart C.J.M. (ed.) (2007). Impacts of climate change on the European marine and coastal environment: ecosystems approach.ESF Marine Board Position Paper. European Science Foundation, Marine Board: Strasbourg, France. 82 pp.
- ↑ Kedra, M.; Walkusz, W. (2006). Global warming-driven biodiversity change: pelagic versus benthic domain [Arctic 79°N case study] MarBEF Newsletter 5: 23-24.
- ↑ Essink, K.; Dettmann, C.; Farke, H.; Laursen, K.; LuerBen, G.; Marencic, H.; Wiersinga, W. (Eds). Wadden Sea Quality Status Report 2004. Wadden Sea Ecosystems 19-2005, 155-161pp. cit. in: Phillipart C.J.M. (ed.) (2007). Impacts of climate change on the European marine and coastal environment: ecosystems approach.ESF Marine Board Position Paper. European Science Foundation, Marine Board: Strasbourg, France. 82 pp.
- ↑ Orr, J.C.; Fabry, V.J.; Aumont, O.; Bopp, L.; Doney, S.C.; Feely, R.A.; Gnanadesikan, A.; Gruber, N.; Ishida, A.; Joos, F.; Key, R.M.; Lindsay, K.; Maier-Reimer, E.; Matear, R.J.; Monfray, P.; Mouchet, A.; Najjar, R.; Plattner, G.-K.; Rodgers, K.B.; Sabine, C.L.; Sarmiento, J.L.; Schlitzer, R.; Slater, R.D.; Totterdell, I.J.; Weirig, M.-F.; Yamanaka, Y.; Yool, A. (2005). Anthropogenic ocean acidification over the twenty-first century and its impact on calcifying organisms. Nature (Lond.) 437(7059) : 681-686.
- ↑ McLachlan, A.; Brown, A.C. (2006). The ecology of sandy shores. 2nd. Edition. Academic Press: Amsterdam, The Netherlands. 373 pp.
Please note that others may also have edited the contents of this article.
|