Rocky shore morphology
Morphology [1]
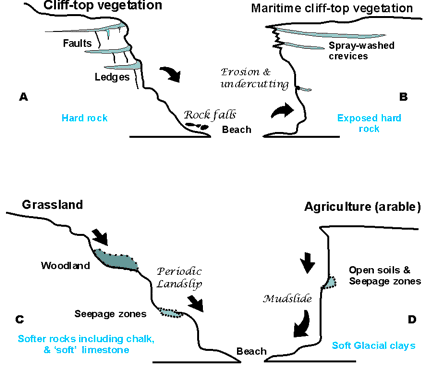
A cliff is defined in the Oxford English Dictionary as "a steep rock face, especially at the edge of the sea". A more precise characterization of sea cliffs is a break in slope between 15 degrees and the vertical, variable in height, found at the contact between the land and sea. Sea cliffs owe their existence in some way to erosion by the sea. Form and height depends on the type of rock - 'hard' rock (metamorphic, igneous and sedimentary rocks) and 'soft' rock (earth cliffs). They make up long stretches of coast.
It is estimated that about half of the world's coastline is backed by a cliff[3]. Cliffs exist in very different forms. This diversity depends, among other things, on mineralogy, lithology, tectonic history, climate, waves and tides. Some rocky coasts consist of steep plunging cliffs; this is observed when subaerial erosion is minor compared to marine erosion or in cases of volcanic activity or tectonic subsidence.
Cliffs which are degraded by subaerial weathering processes are more gently sloping. Such more rounded (often vegetated) cliffs, are called “bluffs”. A common coastal cliff morphology is the slope-over-wall profile: a rounded weathered top and a vertical wall below.
In many cases cliffs are fronted by shore platforms, which can be tens to many hundreds (or even thousands) of meters wide. These shore platforms result from cliff retreat; the outer edge marks the cliff position in the past – often millennia ago, in the case of erosion-resistant rock.
Wave attenuation on the shore platform reduces cliff recession rates[4]. Cliff and shore platform both retreat by erosional processes; the rate of cliff retreat strongly depends on the width of the shore platform. However, shore platforms protruding into the sea also focus wave energy by wave refraction, which can partly offset the wave attenuation function. An equilibrium profile is reached when platform retreat and cliff retreat occur at the same pace.
Rocky coasts often have a crenulated planform. Some crenulated coasts were created by the drowning of river valleys by rising sea level during the Holocene. Other crenulated coasts are produced by differential erosion of material that is weaker than in the adjacent headlands. Coastal indentation is limited by feedbacks: wave attenuation in bays, wave refraction towards the bay heads and accumulation of eroded material in bay-head beaches[5].
Cliff erosion
Cliff retreat depends on many factors: rock structure (massive, bedding, faulted, granular), rock lithology (permeability, solubility, hardness, jointing), hydraulic action (exposure to waves more or less loaded with debris, tides, currents) and subaerial weathering (by surface and subsurface runoff, freeze-thaw, chemical processes, biota). Hard rock cliffs retreat typically less than 1 cm/year, whereas soft cliffs (consisting of boulder clay, for example) retreat typically more than 1 m/year[4].
Cliff retreat is often episodic, through rock-fall and toppling, after undercutting of the cliff base by wave quarrying, abrasion and weathering. Wave quarrying is the process of rock dissection by breaking waves, which produce shock pressures and air compression in joints and fissures[6]. Wave abrasion is the process of rock scraping and shattering by abrasive material (rock fragments) moved forth and back by wave-orbital motion. Rock weathering is due to the mechanical action of alternate drying and wetting and salt crystallization in fissures, but also due to chemical processes (dilution) and biotic activity. Shales and mudstones are particularly sensitive to weathering by repeated wetting and drying. Chalk, limestone, and sandstones are broken down by repeated immersion in saline water[5]. Examples of biotic activity include grazing by limpets[7] and boring by sea urchins, in particular on limestone rock[6].
The cliff top is degraded by surface water running down cliff faces and by groundwater exfiltration that wash material down slope. Groundwater causes loading and lubrication in discontinuities, thus reducing the strength of rock materials. Infiltration of rainwater in soft rock causes suction loss and tension cracks, reducing the stability of the cliff face[8]. Soft-rock cliffs may collapse by slumping or sliding events, which are often preceded by soil creep and which are triggered by rainwater infiltration. Debris fans at the cliff base after a slumping event are subsequently reworked by wave action; they are washed away or become part of the shore platform. Marine dissolution processes are an important cause of degradation of limestone cliffs, when acidic seawater (containing dissolved CO2) and salt spray enter cracks, joints and fissures. Bio-erosion (by algae and by boring and grazing organisms) also contributes to erosion of calcareous rock[6]. The resulting forms are termed “karst”, featuring caves, arches and stacks.
Shore platforms [9]
Shore platforms can be either approximately horizontal, with a steep seaward edge, or gently seaward sloping. Tides modulate the frequency and intensity of wave attack at different levels of the shore platform. Sloping platforms (typically 1 degree to approximately 5 degrees) prevail under macrotidal conditions and strong wave attack, whereas horizontal platforms are more common on micro-tidal coasts. The width of near-horizontal platforms varies between only a few meters to hundreds of meters. Platform elevation also varies considerably, with some platforms formed around low-tide level, others at a range of intertidal elevations, and yet others at a range of supratidal elevations up to several meters above high tide[5]. High-tide terraces are typical for hard-rock coasts and low waves, low-tide terraces for limestone rocks. In the latter case, erosion resistance of the platform surface can be enhanced by carbonate precipitation and other cementing agents (silica, iron). Soft cliffs often have a beach at the base, with an intertidal veneer of sand and gravel. The seaward edge of shore platforms is much less subjected to eroding processes than the cliff base; the seaward edge is generally considered stationary[4]. However, fast retreat of the cliff base generates a wide shore platform, increased platform down-wearing and reduced wave attack at the cliff base. Trenhaile (2020[10]) therefore suggested that the shore platform may adopt an equilibrium slope, consistent with results of cosmogenic isotope analysis of the sandstone shore platform in North Yorkshire, UK[11].
Several processes contribute to erosion of shore platforms. The rate of platform down-wearing is strongly variable from site to site; observed down-wearing rates fall in the range of 0.035 - 1.36 mm/year[5]. Attack by waves armoured with rock fragments is an effective erosion mechanism, which polishes the platform surface. However, large amounts of abrased material and large rock clasts may have a protecting function instead. Part of the abrased material can form a sandy veneer at the cliff base. The finest fraction is washed away by waves and currents.
Platform surfaces usually present many irregularities, with gullies, ponds and potholes scoured in faults, joints and in excavations of less resistant rock around hard outcrops. Chemical and physical weathering plays an important role, through the effects of alternate wetting and drying, salt crystallization and frost. Marine organisms (see Rocky shore habitat) accelerate platform erosion by grazing activity (surface scraping by gastropods) and by boring of holes (e.g. by cyanobacteria, sponges, bivalves, sea urchins). Plant roots can widen crevices and dislodge rock fragments. However, biota can also provide protection against erosion. Seaweeds (e.g. kelp), barnacles and mussels contribute to limiting the impacts of physical weathering agents on shore platforms via moisture retention and buffering effects on temperature variations, wetting-drying, and salt crystallization[12].
A beach of sand or pebbles is often present at the cliff base and on the highest part of the shore platform. This sedimentary deposit is produced by local cliff erosion, as coastal transport is usually small due to the presence of headlands and rocky outcrops. Cliff beaches are narrow, but wide pocket beaches can occur in sheltered zones between headlands. Sediments are usually coarse and poorly sorted, and can consist of sand and wave-rounded clasts, as well as angular debris, ranging to large boulders in many regions[13].
Related articles
References
- ↑ Trenhaile, A.S. 2011. Cliffs and Rock Coasts. In: Treatise on Estuarine and Coastal Science Vol. 3, eds. Flemming, B.W. and Hansom, J.D., Elsevier, p. 171-192
- ↑ Carter, R.W.G. 1988. Coastal Environments. An Introduction to the Physical, Ecological and Cultural Systems of Coastlines. Academic Press, London.
- ↑ Young, A.P. and Carilli, J.E. 2019. Global distribution of coastal cliffs. Earth Surface Processes and Landforms 44: 1309–1316
- ↑ 4.0 4.1 4.2 Sunamura, T. 1992. Geomorphology of Rocky Coasts. Wiley, Chichester
- ↑ 5.0 5.1 5.2 5.3 Stephenson, W.J., Dickson, M.E. and Trenhaile. A.S. 2022. Rock coasts. Chapter 8.19, Treatise on Geomorphology 2nd edition. Elsevier
- ↑ 6.0 6.1 6.2 Trenhaile, A.S., 1987. The Geomorphology of Rock Coasts. Oxford University Press, 384 pp.
- ↑ Andrews, C. and Williams, R.B.G. 2000. Limpet erosion of chalk shore platforms in southeast England. Earth Surface Processes and Landforms 25: 1371–1381
- ↑ Brooks, S.M., Spencer, T. and Boreham, S. 2012. Deriving mechanisms and thresholds for cliff retreat in soft-rock cliffs under changing climates: rapidly retreating cliffs of the Suffolk coast, UK. Geomorphology 153: 48–60
- ↑ Bird, E.C.F. 2008. Coastal Geomorphology: An Introduction, Second Edition. JohnWiley and Sons, Ltd, pp. 411
- ↑ Trenhaile, A.S. 2020. Modelling the development of dynamic equilibrium on shore platforms. Marine Geology 427, 106227
- ↑ Swirad, Z.M., Rosser, N.J., Brain, M.J., Rood, D.H., Hurst, M.D., Wilcken, K.M., Barlow, J., 2020. Cosmogenic exposure dating reveals limited long-term variability in erosion of a rocky coastline. Nature Communications 11, 3804
- ↑ Gonzalez, J.A., Coombes, M.A., Palomo, M.G., Isla, FI., Soria, S.A .and Gutiérrez, J.L. 2021. Enhanced Weathering and Erosion of a Cohesive Shore Platform Following the Experimental Removal of Mussels. Front. Mar. Sci. 8:756016
- ↑ Trenhaile, A. 2016. Rocky coasts ― their role as depositional environments. Earth-Science Reviews 159: 1-13
Please note that others may also have edited the contents of this article.
|