Marine Plankton
Definition of Plankton:
Assemblage of aquatic organisms, usually microscopic, that drift passively or, if they swim, are generally unable to move against the prevailing currents. These include bacteria and microalgae (phytoplankton), microscopic crustacea, cnidarians, larvae of fish and other animals (zooplankton). They constitute the base of the aquatic food chains[1].
This is the common definition for Plankton, other definitions can be discussed in the article
|
Contents
Introduction
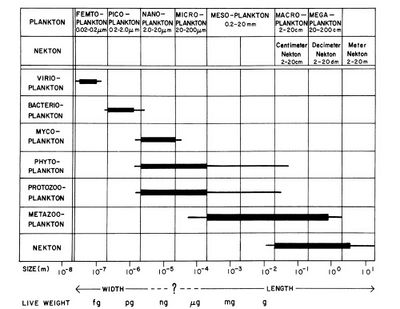
Plankton consists of a diverse range of living organisms that spend at least a part of their life cycle suspended in water. The term plankton is actually a Greek word, meaning that which is made to wander or drift. This term is further divided into the phytoplankton and zooplankton, meaning plant- (Gk. phyto) and animal- (Gk. zoön) drifters respectively.
Planktonic organisms are largely at the mercy of oceanic currents and water movements with only little ability to control their fine-scale distribution in the water column. Holoplankton refers to those organisms that spend their entire life in the plankton, as opposed to the meroplankton, which are only planktonic for a part of their lives. Organisms that are capable of resisting the powers of currents, such as fish and squid, are referred to as nekton.
Planktonic organisms are typically classified into broad size categories according to the 'Sieburth-scale' (Fig. 1), originally proposed in 1978. Viruses and jelly fish sit at opposite ends of this scale, which runs from fractions of a millimetre to metres. Most planktonic species are microscopic in size. Picoplankton is on average ten times more abundant than nanoplankton in the ocean and a thousand times more abundant than microplankton. However, the size classes of picoplankton and nanoplankton represent similar particle surface concentrations, and thus contribute similarly to the light scattering and absorption properties of seawater. The volume concentration of nanoplankton in the ocean is on average significantly greater than the volume concentration of microplankton and picoplankton, except in Arctic waters where the volume concentrations of nanoplankton and microplankton are comparable[3].
A classification of marine microbial organisms is shown in the article Marine microorganisms.
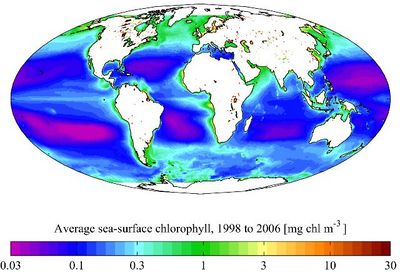
Phytoplankton
Marine ecosystems, which are the major backbone of life on earth and constitute the largest source of habitat and biodiversity, are primarily supported by phytoplankton, also called microalgae. They provide the basic food in the food web/chain and play an important role in nutrient recycling and gaseous exchange.
Marine phytoplankton are taxonomically diverse and comprise various groups, including diatoms, coccolithophores, chlorophytes, flagellate protists (dinoflagellates, raphidophytes) and cyanobacteria. In total, it consists of about 5000 extant species as a whole. Photosynthesis is the process by which phytoplankton combines inorganic building blocks such as carbon dioxide (CO2) and water (H2O) to produce organic compounds, using energy from the Sun. Organisms that are capable of this process are referred to as photoautotrophs (Gk. Photon, auto, troph; light-self-nutrition) and primary producers. Chlorophyll and other similar pigments are found in light-harvesting organelles called chloroplasts. These are present in all species of phytoplankton. Organic matter formed by these organisms forms the basis of almost all food chains. Approximately 50% of global primary production occurs in the oceans. When the water temperature is favorable and light and nutrients are sufficiently available, algal colonies can grow exponentially and form huge blooms that collapse after depletion of the nutrient pool, see Algal bloom dynamics. Physico-chemical parameters including light, nutrients, density stratification and turbulence are major factors influencing spatial and temporal variations of phytoplankton.
On land, plants are typically large, conspicuous organisms; trees, herbs, bushes and grasses etc. They have root structures to take up water and nutrients from the soils beneath them, and also to provide anchorage. Phytoplankton are fundamentally different. They are microscopic, single-celled organisms. Smaller objects have a greater surface area relative to their volume, and hence mass. Being small in the oceans confers several advantages: Phytoplankton cells are so small that their weight is, to a large extent, offset by the frictional drag exerted on them by the water. Thus, they sink very slowly, enabling them to stay within the surface, sunlit waters. Phytoplankton species often have ornate spines and appendages which increase drag, and serve as defence mechanisms. Many plankton species also use a chemical defence against their predators, either through toxin production or feeding deterrence, see Chemical ecology. Phytoplankton cells do not have roots, and must take up nutrients from their surrounding environment. Having a large surface area relative to their volume ensures that they maximise their chances of attaining enough resources for growth.
Pelagic environments are usually classified according to their levels of plankton chlorophyll-a (chl-a) as oligotrophic (chl-a < 0.1 mg/m3), mesotrophic (0.1 < chl-a < 1 mg/m3), and eutrophic (chl-a > 1 mg/m3), which is approximately equivalent in terms of primary production to < 50, 50—200, and > 200 gC/m2 per annum. In addition, most pelagic plankton belong to one of the three major functional size classes: picophytoplankton (0.2—2.0 μm), nanophytoplankton (2—20 μm), and micro-phytoplankton (20—200 μm) [4]. The oceans are mostly oligotrophic, while most coastal seas are eutrophic (Fig. 2).
Picophytoplankton accounts for a significant fraction of primary production in seawater, especially in oligotrophic environments. This group includes the cyanobacteria Prochlorococcus and Synechococcus, which are the most abundant photosynthetic organisms on Earth[5]. Prochlorococcus typically dominate oligotrophic environments, whereas Synechococcus and larger-sized phytoplankton tend to be more abundant under mesotrophic conditions. Many heterotrophic bacteria and some protists also fall in the picophytoplankton size class. Heterotrophic bacteria either absorb organic matter directly from their surroundings, or actively capture and ingest bacterial prey. They are generally the most abundant component of the picophytoplankton and major contributors in the cycling of carbon and nutrients (see Nutrient conversion in the marine environment). Other bacteria are capable of both autotrophic and heterotrophic nutrition: they are mixotrophic.
Nanophytoplankton include most species of flagellates, autotrophic, heterotrophic, and mixotrophic, along with some smaller-sized non-flagellated green algae and diatoms, and the smallest species of dinoflagellates and ciliates. Heterotrophic nanoflagellates are dominant consumers of picophytoplankton and important remineralizers of organic matter and nutrients[4].
Micro-phytoplankton are microscopic photosynthetic creatures which exist in different forms (single, filamentous or colonial), shapes (ovoid, cylindrical, spherical) and size. They encompass the larger-sized phytoplankton, mainly single cells and chains of diatoms, larger species of photosynthetic dinoflagellates, and larger-sized phagotrophic protists, ciliates, and heterotrophic dinoflagellates.
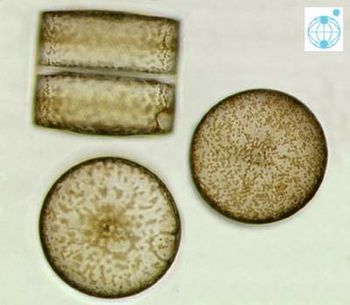
Diatoms
Perhaps the most conspicuous group of phytoplankton are the diatoms (Fig. 3). Their name is derived from the Greek words dia and temnein, literally cut in half. This seemingly bizarre name arose because of the nature of their cell wall, or frustule, which is made up of two halves or valves like that of a laboratory petri dish. The silicon-rich frustule is perforated by numerous pore-like structures which can give the cell a beautiful appearance when viewed under high magnification. These connect the cell to the outside seawater. They also help strengthen the cell wall whilst reducing its mass. Diatoms can regulate their buoyancy via the biosynthesis of lipids (triacylglycerols), which are more lightweight than other chemical constituents of these organisms. As these lipids are rich in energy, diatoms have great potential as a biofuel source[7].
Diatoms tend to be dominant in eutrophic environments, often referred to as “classical” marine food webs. It has been shown that their cell cycle is interrupted under silicon-limited conditions. In temperate latitudes, diatoms are well known for their capacity to form immense blooms in spring as the sun’s energy infiltrates nutrient rich surface waters. Diatoms require silicon for cell division because of the unique structure and morphogenesis of their frustule. Their rapid growth rates are thought to confer them a competitive advantage during these times, only to be thwarted as silicate concentrations become depleted. Diatoms produce large quantities of mucus when nutrient stressed, causing them to coagulate and sink into the deep ocean. The ecological significance of this is still not fully understood as it appears to represent a genetic dead-end i.e. once in the deep sea, they do not possess a mechanism to enable them to return to the surface. Diatoms are one of the major producers of polyunsaturated fatty acids, which are now widely reputed as being beneficial for human health. Diatoms are a good food source for herbivores, but some species produce chemicals (oxylipins) as a defence against being grazed, see Functional metabolites in phytoplankton. Diatoms of the genus Nitzschia produce a neurotoxin (domoic acid). Consumers of shellfish that have been exposed to this toxin suffer from Amnesic Shellfish Poisoning (ASP), see Harmful algal bloom.
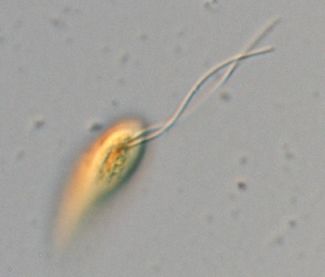
Flagellates
Much of the remainder of marine plants belong to a diverse assemblage of unicellular organisms called flagellates (Fig. 4). These cells possess a whip-like flagellum. It is at this end of the plankton size spectrum that the distinction between plant and animal becomes blurred. The flagella are used to propel the cell, enabling them to ‘swim’ upwards towards the sunlit waters. Some flagellates, although mobile, contain photosynthetic pigments and are thus autotrophic. Others are devoid of pigments and hence are heterotrophic. Mixotrophy, blurring the distinction between phytoplankton and zooplankton, is very common among flagellates [8].

Dinoflagellates
Dinoflagellates are comparatively large flagellates. They are so-called because of the way that they ‘whirl’ or corkscrew through the water (Gk. Dinos; whirling). Approximately half of all the dinoflagellates are autotrophic and those belonging to the other half are either heterotrophs or mixotrophs (see below). Many armour their cell wall with cellulose plates, forming the theca (Fig. 5). In some species of thecate dinoflagellates the plates form long spines which serve to increase the cell’s frictional drag and also act as a grazing deterrent. The ‘naked’ dinoflagellates lack any such extracellular armour.
Several species of dinoflagellates bioluminesce: They produce a flicker of light when disturbed. This is frequently observed by SCUBA- (Self Contained Breathing Apparatus) divers at night when they turn off their torches and disturb the water with their hands. Some species of dinoflagellates can produce virulent neurotoxins such as saxitoxin and brevetoxin. These can cause serious harm if they enter the human food chain. For this reason, monitoring programmes exist to ensure that the fish and shellfish that we consume are safe, see Harmful algal bloom.
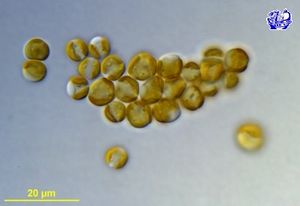
Phaeocystis
Phaeocystis (Fig. 6) is a particularly interesting genus of flagellate. It can exist as small, individual cells or large gelatinous colonies. The latter are long since known for fouling fishermen’s nets. Large blooms of these organisms can occur throughout the world, particularly in the temperate and polar seas. These are problematic for fish as the gelatinous colonies can clog their gills. Indeed, blooms of Phaeocystis can trigger dramatic changes in the structure of marine ecosystems owing to its seemingly unpleasant nature. It produces a strong smelling compound called dimethylsulfupropionate (DMSP) which is thought to serve as a grazing deterrent. It is also suggested to play a role in climate regulation by stimulating the formation of clouds. There is growing concern that the frequency and magnitude of Phaeocystis blooms in coastal waters are increasing as a result of eutrophication. See also Foam beach, Sydney.
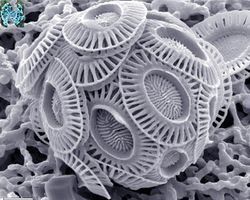
Coccolithophores
Coccolithophores (or coccolithophorids, Fig. 7) are another important group of flagellates. Each cell is covered by an array of intricate plates or coccoliths (the coccosphere) made from calcium carbonate. Coccolithophores are the most prolific calcifying organisms on Earth. The exact function of the coccoliths is unknown. The coccoliths have been suggested to serve as a grazing deterrent, to help maintain buoyancy and to act as an ultra-violet radiation filter. Certain species produce enormous blooms that are clearly visible from space, causing the water to have a milky-white appearance that fishermen refer to as ‘white water’.
The production of calcium carbonate by coccolithophores also plays a role in global warming. On the one hand, by lowering the ocean surface alkalinity, dissolved CO2 is released back into the atmosphere. On the other hand, coccoliths provide a sink for emitted carbon to the ocean floor, mitigating the effects of greenhouse gas emissions (see also Ocean acidification).
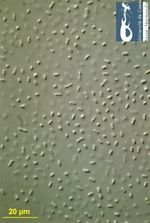
Cyanobacteria
Cyanobacteria, or the blue-green algae (Fig. 8), play a unique role in the nutrient-depleted waters of the open oceans. They are capable of converting inert atmospheric nitrogen (N2) into organic forms such as nitrate (NO3-) and ammonia (NH3). This process is known as nitrogen fixation. It provides a valuable source of nitrogen in areas of the oceans where it is otherwise absent.
Zooplankton
The extremely diverse collection of organisms referred to as zooplankton is all but hidden from the public eye. It is presumably for this reason that some of the most numerous animals on our planet remain without common names. Every major phylum of the animal kingdom (except the insects) is represented in the zooplankton, at least by a larval stage. Zooplankton plays a crucial role in the pelagic food web as link between primary producers and higher trophic levels.
Protozoa
Protozoa (Gk. Proton, zoön; first-animal) is a loosely-defined collection of unicellular, heterotrophic organisms. Together with small multi-cellular organisms they form the microzooplankton. Marine protozoans include ciliates, foraminiferans, radiolarians and some dinoflagellates. As noted above, the distinction between plant and animal becomes blurred as we travel down towards the smaller end of the plankton size spectrum.
In estuaries, microzooplankton can graze up to 60% of the daily primary production and thus exerts strong control over the phytoplankton community. In addition, the high lipid content of microzooplankton and their ability to buffer nutritional imbalances renders them high quality food items for mesozooplankton and newly hatched fish larvae[9]. In fact, microzooplankton is often a primary food source for mesozooplankton, bypassing the direct link between phytoplankton and copepods[10].

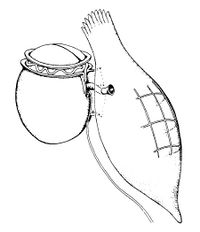
Ciliates (Fig. 9) are a particularly important group of protozoa. As their name suggests, they possess numerous hair-like features that fulfil several functional roles including movement, feeding and sensing the external environment. Heterotrophic ciliates focus on prey much smaller than themselves, ingesting bacteria and/or nanoflagellates. They play key roles in the microbial loop (see below).
Heterotrophic dinoflagellates frequently attack prey of equal or larger size than themselves (Fig. 10). Some feed by engulfing their prey whole, while others suck out the contents of their prey via a feeding appendage called the peduncle.
Crustacea
Planktonic crustaceans are in many ways analogous to the insects on land. They typically dominate zooplankton communities, representing a crucial link in oceanic food chains. The term crustacean is derived from the Latin word “crustaceus”, meaning ‘having a shell or crust’. This refers to the jointed armour that envelops their bodies, made from a tough material called chitin (Gk. chiton, a tunic). This rigid external skeleton restricts the growth process, and must first be shed before an individual can increase in size. Growth and development of all crustaceans is therefore achieved through a series of moults. It is common for the body form of an adult to be considerably different to that of the young.
Copepods. In the words of Sir Alister Hardy, the world-renowned zooplankton biologist, “the crustaceans of the marine plankton par excellence are the Copepoda” [12]. The abundance and biomass of copepods (Fig. 11) in our oceans defies belief. Some people maintain that copepods are the most numerous animal on our planet. They are found in all the seas on Earth, and have developed numerous strategies to survive in even the harshest conditions. Copepods may be herbivorous, omnivorous, carnivorous or even detritivorous. They typically have an array of specialised feeding appendages that enable them to effectively sieve-out or grasp their food from the surrounding water. It is these oar-like feet that give copepods their name (Gk. Kope, Podo; oar-foot). The sheer numbers of these organisms necessitates that they play major roles in the ecology of our seas. Copepods are often the first prey of fish larvae, so a healthy population of these tiny creatures is essential for healthy fish stocks.
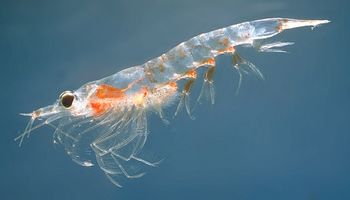
Euphausids are relatively large (10-50 mm) shrimp-like animals that are more commonly known as krill (Fig. 12). They are thought be omnivores, filtering out phytoplankton and similar-sized microzooplankton from seawater. Krill are often found in large swarms. This is thought to confuse predators that are searching for an individual. Euphausids (Gk. Phausis; Shining light) are so-called because they have light-producing organs. Exactly why they produce light remains unknown. It may be involved in mate-location, social interaction or camouflage. Krill are super-abundant, particularly in the polar seas. They represent a staple food source for a diverse array of animals, ranging from fish and penguins to seals and whales.
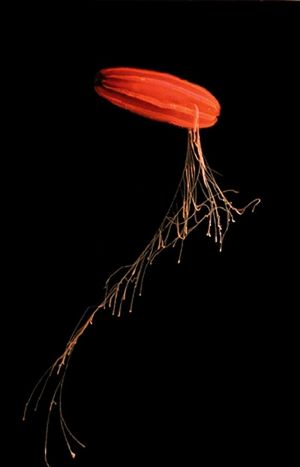
Amphipods (Fig. 13) are another important type of planktonic crustacean. They have large (sometimes enormous), well-developed eyes at the front of their head. These, together with pincer-type feeding appendages, are used to actively seek-out and capture their prey. The striking appearance of planktonic amphipods is reputed to have inspired the form of the creature in Ridley Scott’s 1979 film ‘Alien’. Some amphipods have a tendency to swarm like krill, whereas others live in association with gelatinous organisms such as jellyfish and salps. Upper ocean amphipods are eaten by an array of larger animals, including fish, birds and marine mammals. Deep water amphipods are important scavengers, and are always amongst the first animals to arrive when a new food source becomes available.
Many crustacean zooplankton undertake a daily migration, moving into the upper, food-rich waters at night, and descending into the inky depths during the day. This behaviour continues throughout the winter, despite the surface layers being devoid of food. It is thought that ‘diel vertical migration’ is a tactic to avoid predators that feed visually.
'Jellies'
The oceans harbour an enormous array of gelatinous organisms, or ‘jellies’ , that range in size from millimetres to meters. They have soft, translucent and often fragile bodies. The latter makes them notoriously difficult to sample in a quantitative manner. This has led to the significance of gelatinous organisms in marine ecosystems being underestimated historically. A common feature of all jellies is that they are carnivorous, although some contain endosymbiotic algae that provide sugars and other carbohydrates via photosynthesis.
Jellyfish are in fact not fish at all. They belong to a collection of organisms known as cnidarians. They are distinguished by the presence of nematocysts or cnidocytes], which are stinging cells. These serve as efficient weapons, firing tiny dart-like structures that deliver neurotoxins into their prey. Jellyfish are known to form large blooms. This largely reflects their seasonal reproductive efforts. It has been suggested that the frequency and magnitude of jellyfish blooms has increased as a result of overfishing. However, there are currently too few data to substantiate or refute this claim. Jellyfish are consumed by other jellyfish, as well as fish and turtles.
Ctenophores (Fig. 14) are distinct from jellyfish because they do not possess cnidocytes. They are more typically known as the comb jellies (Gk. kteis, ktenos; comb), owing to the 8 rows cilia which have a comb-like appearance. The rhythmic beating of these tiny hairs provides propulsion for these animals. The cilia refract light like a prism, giving rise to wave upon wave of rainbow colours that sweep over their body. In the 1980’s an invasive American species of ctenophore was accidentally introduced into the Black Sea via a ship’s ballast water. The introduced ctenophore became the dominant consumer of zooplankton, removing the prey items for fish larvae. Commercial fisheries went into decline as a result of this invasive species, causing massive socio-economic disruption in the region.
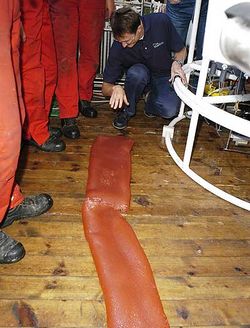
Salps, doliolids, pyrosomes and larvaceans are pelagic tunicates. They range in size from a few millimetres to metre-long colonies (Fig. 15). Typically they have a barrel-shaped body that has two openings – one for taking water in, and the other for allowing water to be pumped out. Muscular contractions and rhythmic beating cilia draw water over a sticky mucus basket that sieves out plankton as it is pumped through the animals body. The larvaceans surround their bodies with a thin gelatinous ‘house’ which is periodically shed as the animal grows.
Chaetognaths
The chaetognaths (L. chaeta, a bristle; Gk. gnathos, jaw) are a particularly interesting group of zooplankton. They are commonly known as the ‘arrow-worms’ because of their slender arrow-like nature. Every ocean of the world contains a chaetognath representative, and they are typically amongst the commonest animals encountered in the plankton. Arrow worms are voracious predators, with curved bristles that act as powerful jaws. They hunt by remaining motionless in the water until an unsuspecting copepod moves within striking distance; then they dart forward and grasp their prey. It is easy to overlook arrow worms in live plankton samples because they are almost completely transparent, often only becoming visible when they move.
Microbial loop
Some of the organic molecules produced by phytoplankton are exuded as dissolved organic matter (DOM). Similarly, DOM is released due to ‘sloppy feeding’ as zooplankton graze on phytoplankton cells. Each millilitre of seawater contains approximately 1 million bacterial cells, many of which utilise DOM as a source of energy and nutrition. The abundance of these bacteria is, to a large extent, regulated by the grazing effects of heterotrophic nano-flagellates (2 – 20 μm in diameter). In turn, the heterotrophic nanoflagellates are grazed by larger heterotrophic organisms such as dinoflagellates and ciliates. Thus, DOM released by primary producers is channeled back into the food chain by what is known as the ‘microbial loop’.
Sampling the plankton
All planktonic organisms were traditionally sampled with a fine-mesh net, the exact size and nature of which depending on the target organisms. Silk, such as the ‘bolting cloths’ originally used by millers to sieve flour, was used for net manufacture prior to the advent of nylon meshes. Plankton nets are typically conical in shape. They are towed or hauled through the water, funneling organisms towards the ‘cod end’ where they remain trapped.
Phytoplankton
Contemporary studies interested in the vertical distribution of phytoplankton species typically collect seawater samples with Niskin bottles. The constituent phytoplankton cells are subsequently preserved with Lugol’s iodine for later examination with a microscope. The chlorophyll content of seawater is frequently used as an indicator of phytoplankton abundance and biomass. Broad-scale (km) surveys of chlorophyll can be achieved by towing a fluorimeter through the water. The colour of the surface of our oceans can now be accurately measured from space [14]. These ‘ocean colour’ measurements enable global-scale monitoring phytoplankton growth patterns.
Zooplankton
Modern biological oceanographers have a suite of nets and sampling arrays to help them collect and count zooplankton from discrete depths of the ocean. Broad-scale surveys can be undertaken by towing an optical plankton counter (OPC) behind a research vessel. Zooplankton are funneled into a narrow corridor and through a beam of light. A computer counts the number of times that the beam of light is broken, and thus the number of zooplankton. The larger types of zooplankton reflect sound and can thus be surveyed by using acoustic techniques. This type of survey uses techniques identical to those used by fishermen to locate fish. See Sampling tools for the marine environment for an overview of other sampling equipment.
The development of the Continuous Plankton Recorder (CPR), by Sir Alistair Hardy and colleagues in the 1920’s and 30’s, represents one of the major milestones in plankton biogeography [15]. Each CPR is a self-contained sampling device that collects animals on to an array of ‘silks’. They are designed to be deployed by merchant ships crossing the oceans (Fig. 16). This enables a far greater number of observations than would be achievable using dedicated research vessels alone. The CPR survey, which now spans 75 years, has revolutionized our understanding of the distribution and seasonality of plankton.
Related articles
- Marine microorganisms
- Algal bloom dynamics
- Plankton remote sensing
- Plankton remote sensing North Sea
- Differentiation of major algal groups by optical absorption signatures
- Remote sensing of zooplankton
- Light fields and optics in coastal waters
- The Continuous Plankton Recorder (CPR)
- In situ monitoring of eutrophication
- Sampling tools for the marine environment
- Harmful algal bloom
- Chemical ecology
- Functional metabolites in phytoplankton
References
- ↑ Barnes, R. and Hughes, R. 1999. An introduction to marine ecology. Wiley & Sons, Oxford
- ↑ Sieburth, J. Mn., Smetacek, V. and Lenz, J. 1978. Pelagic ecosystem structure: Heterotrophic compartments of the plankton and their relationship to plankton size fractions. Limnology and Oceanography 23: 1256-1263
- ↑ Reynolds, R. A. and Stramski, D. 2021. Variability in oceanic particle size distributions and estimation of size class contributions using a non-parametric approach. Journal of Geophysical Research: Oceans 126, e2021JC017946
- ↑ 4.0 4.1 Kämpf, J., Newman, M., Doubell, M., Möller, L., Baring, R., Shute, A. and Redondo Rodriguez, A. 2021. A study of the seasonal and interannual variability of phytoplankton and zooplankton assemblages in a significant marine ecosystem. Oceanologia 65: 434—451
- ↑ Flombaum, P., Gallegos, J.L., Gordillo, R.A., Rincón, J., Zabala, L.L., Jiao, N., Karl, D.M., Li, W.K.W., Lomas, M.W., Veneziano, D., Vera, C.S., Vrugt, J.A. and Martiny, A.C. 2013. Present and future global distributions of the marine Cyanobacteria Prochlorococcus and Synechococcus. PNAS 110: 9824-9829
- ↑ 6.0 6.1 6.2 6.3 6.4 6.5 6.6 Plankton Net; Data Provider at the Alfred Wegener Insitute for Polar and Marine Research: http://planktonnet.awi.de/
- ↑ Stonik, V. and Stonik, I. 2015. Low-Molecular-Weight Metabolites from Diatoms: Structures, Biological Roles and Biosynthesis. Mar. Drugs 13: 3672-3709
- ↑ Flynn, K.J., Stoecker, D.K., Mitra, A., Raven, J.A., Glibert, P.M., Hansen, P.J., Graneli, E. and Burkholder, J.M., 2013. Misuse of the phytoplankton-zooplankton dichotomy: the need to assign organisms as mixotrophs within plankton functional types. J. Plankton Res. 35: 3–11
- ↑ Stoecker, D. and Pierson, J. 2019. Predation on protozoa: its importance to zooplankton revisited. J. Plankton Res. 41: 367–373
- ↑ Saiz, E. and Calbet, A. 2011. Copepod feeding in the ocean: scaling patterns, composition of their diet and the bias of estimates due to microzooplankton grazing during incubations. Hydrobiologia 666: 181–196
- ↑ Hansen, P.J. and Calado A. J. 1999 Phagotrophic mechanisms and prey selection in free-living dinoflagellates. J. Eukaryot. Microbiol. 46: 382-389
- ↑ Sir Alistair Hardy, 1959. The open sea – its natural history: Part I The world of plankton, 2nd Edition. Readers Union, Collins, London
- ↑ 13.0 13.1 13.2 MAR-ECO: http://www.mar-eco.no/
- ↑ Ocean Colour: http://disc.gsfc.nasa.gov/oceancolor/index.shtml
- ↑ SAHFOS: http://www.sahfos.ac.uk/
Please note that others may also have edited the contents of this article.
|