Coastal mud belt
Accumulation of fine sediments in the nearshore zone occurs when a flow pattern exists on the inner continental shelf that prohibits escape to deeper water. In this regard, a coastal mud belt may be regarded as the coastal equivalent of an estuarine turbidity maximum.
Contents
Mud aggradation on the shoreface
The sediments of beaches on the open sea usually consist of sand or gravel. This also holds for the shoreface that extends underwater to depths of ten meters up to several tens of meters. Fine sediments carried onshore cannot settle in this zone due to seabed stirring caused by shoaling and breaking waves. They usually settle on the inner- or mid-shelf where the seabed is less affected by wave action. They can consolidate to form an erosion-resistant seafloor or be transported further to deep water, often as a nepheloid near-bottom layer that is maintained and kept in movement by oceanic currents, tidal currents or currents generated by internal waves[1]. However, there are circumstances where fine sediment can accumulate in the shoreface zone and even around the shoreline. These conditions are characterized by a large availability of fine sediments and a wave climate dominated by waves with small steepness (in particular swell coasts). In that case, a feedback mechanism can be triggered whereby deposition of fine sediment creates a condition appropriate for ongoing deposition. This mechanism is the damping of incident waves by absorption of wave energy in a bed layer with high mud concentration[2][3] (Fig 1). The absorption of wave energy causes this mud layer to behave like a high-density liquid, a so-called fluid mud layer. A more detailed description of this phenomenon can be found in the article Fluid mud. The absorption of wave energy by the fluid mud layer makes that waves are strongly damped without breaking. They lose their capability to stir up fine sediments settled to the shoreface bed and to carry these sediments offshore to deep water. This allows ongoing deposition of fine sediments in the beach zone. An example of wave damping by fluid mud in the shoreface zone is shown in Fig. 2, from observations in the coastal zone off Kerala (India)[4].
Wave damping generates a stress (so-called radiation stress) in the direction of decreasing wave height. This stress acts on the fluid mud layer by pushing it onshore. Onshore-offshore asymmetry of the wave orbital motion due to nonlinearity of the interaction with the bed (see Shoreface profile) also plays a role in onshore transport.
![]() Fig. 2. Records of significant wave height [math]H_s[/math] off the Kerala coast (India). Black line: wave height at 15 m depth, seaward of a mud bank. Blue line: wave height at the mud bank. Adapted from Samishka et al. (2017[4]). |
Formation of coastal mud belts
Coastal mud belts occur when fine sediments supplied by rivers cannot escape easily to deep water but are confined to a narrow coastal zone (typically some tens of kilometers wide). Upwelling is an important mechanism for limiting the width of the mud zone. Upwelling occurs along coasts where the prevailing winds are directed along the coast, with the coast on the left in the northern hemisphere and on the right at the southern hemisphere. In this case, the upper water layer is deflected offshore due to the effect of earth rotation (see Ekman transport). An onshore directed return flow is established in the lower part of the vertical, see Fig. 3. This return flow conveys nutrient-rich water from the deep ocean to the coastal zone, a phenomenon called upwelling. It also counteracts the downslope motion of fine sediment layers to the deep sea, thus confining fine sediment to a coastal mud belt. Close to the equator, where the Coriolis effect is small, upwelling occurs for winds with an offshore directed component. Upwelling currents have been reported for several mud belt coasts: for the coast of Surinam (Eisma 1966[5]), for the coast of southwest India (Rao et al. 1985[6]) and for the coast of the East China Sea (Hu et al. 1984[7]).
Salinity induced stratification is another mechanism that confines fine sediments to a narrow coastal strip. When a buoyant river plume is bent along the coast (by earth's rotation or wind stress), the nearshore salinity is lower than offshore and the salinity near the surface is lower than near the bottom. The resulting cross-shore and vertical density gradients both contribute to producing net onshore transport in the lower part of the water column. It is likely that the upwelling currents at the northern coast of South America and at the East China coastal zone are largely due to density gradients induced by the river plumes of the Amazon and Yangtze, respectively.
The underlying oceanographic processes have been studied in detail for the onshore transport of fine sediment induced by the Rhine river plume. The Rhine plume follows the Dutch coast on the right hand, in the same direction as the progressive semidiurnal tidal wave (which is well represented by a Kelvin wave). The cross-shore density gradient induces gravitational circulation similar to estuarine circulation [8] (Fig. 4). The vertical density gradient has a tidal component that increases during rising tide and decreases during falling tide. Pycnoclines are more inclined at high water than at low water, which is a consequence of the cyclonic rotation of the tidal velocity vector near the bottom (i.e. with an onshore directed component during rising tide and offshore directed component during falling tide) and the anticyclonic rotation near the surface (see Fig. 5 and Coriolis and tidal motion in shelf seas)[9]. The onshore directed component of the near-bed velocity during rising tide is enhanced by the cross-shore salinity-induced density gradient. This velocity component is thus stronger than the offshore directed near-bed velocity component during falling tide. It raises the turbidity in the Rhine-influenced nearshore zone to a concentration that is about ten times higher than offshore (but not high enough for creating a mud bed - the Rhine has a low suspended matter load)[10][11]. Similar observations of fine sediment trapping along river-influenced coastal zones have been reported by Geyer et al. (2004[12]).
Occurrence of coastal mud belts
Elongated mud belts establish along coasts that have characteristics as described in the previous sections: (1) sufficient availability of fine sediment, (2) a large-scale circulation pattern in which the net flow along the bottom has a landward component and (3) alongshore sediment transport. These coasts are a subcategory of the more general mud coasts, which occur where large rivers discharge sediment with a high mud fraction to the coastal zone. Three large mud belt systems are extensively described in the literature: the mud belt along the northern coast of South America (from the Amazon to the Orinoco delta)[13][14][15][16], the mud belt along the southwestern coast of India (Kerala coast)[17][4][18] and the mud belt along the East China coast (from the Yangtze delta to Taiwan)[19][20]. The three mud belt coasts have developed through similar mechanisms, but in other respects they are quite different; a brief description is given below. There are also mud belts along other upwelling coasts, for example along the coast of West Africa (Senegal[21], Guinea [22], Namibia[23]). However, hydro-sedimentary studies of these coasts are scarce.
Northern coast of South America (Guyanas coast)

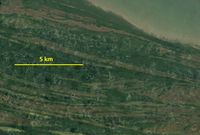
The mud belt along the coast of the Guyanas (Fig .6) is fed with fine sediments discharged by the Amazon river. Part of the mud deposits on the Amazon outer delta is kept mobile due to frequent resuspension induced by waves and tidal currents[24]. The mud is transported in northwestern direction by the North Brazilian Current and further westward along the coast under the influence of the easterly trade winds and obliquely incident waves[25]. Onshore directed bottom currents, induced by surface wind stress and cross-shore salinity gradients, confine the mud in a coastal strip of about 40 km wide (water depths between 0 and 20 m) [5]. The alongshore mud transport has an intermittent character with an estimated cyclicity of about 30 years[26]. This intermittency (which is not yet well explained) is associated with the presence of large mud banks of up to 5 m thick and 10-40 km long that migrate over a bed of relict consolidated mud[14]. The 1400 km long mud belt of the Guyanas coast totals some 20-25 mud banks.
Strong coastal accretion occurs upon the arrival of the leading edge of a mud bank (up to a few hundreds of meters) through onshore transport of fluid mud by nonlinear wave-mud interaction processes[15]. Fluid mud can form from liquefaction of an unconsolidated mud bed by cyclic wave-induced loading[27], but it more generally results from the collapse of a suspended high-concentration mud layer (pers. com. Han Winterwerp). Waves can lose more than 90% of their energy by viscous damping in the fluid mud[28], thus allowing for mud deposition on the intertidal beach zone. The fresh mud deposits are rapidly colonized by mangroves which promote further mud accretion. After the passage of the mud bank (the interbank phase), waves are no longer damped by fluid mud. This results in strong erosion at the trailing edge of the mud bank and during the interbank phase[15] (Fig. 7).
During the erosion process, fine sediments are winnowed from the unconsolidated mud deposit, leaving ridges of sandy and shelly material[29]. These so-called cheniers are transported inland during the interbank period, as a result of swash-induced transport over the indurated mud bed (Fig. 8).
Coast of southwest India (Kerala)
Along the swell coast of Kerala a belt consisting of several separate mud banks extends over a length of about 50 km in the nearshore zone in the depth range of 3 – 30 m (Fig. 9). The mud banks are activated in the monsoon period, when high waves from the southwest stir up the mud deposits, generating fluid mud patches that absorb a large part of the wave energy (Fig. 2). The dimensions of the fluid mud patches vary between 2 and 5 km in the alongshore direction and 1.5–4 km in the offshore direction[18]. The origin of the mud deposits is not well identified but probably not recent, as most of the current fluvial sediment load is retained in upstream reservoirs and downstream lakes[4]. It has been suggested that the pattern of mud patches has a self-organized origin[15], possibly through enhanced deposition in areas where turbulence is suppressed by the presence of embryonic mud patches[30] or through ‘’streaming’’ by dissipating waves that refract towards the mudbanks.
The offshore escape of the sediment is prohibited by upwelling currents induced by monsoon winds directed to the south along the Kerala shoreline[31][32]. The wave attenuation in the mudbank area generates gradients in the longshore drift, similar to the effect of an offshore breakwater, causing beach accretion in the sheltered zone and beach erosion at the downdrift side[18]. Mud does not accumulate on the beaches, which are sandy. Some fluid mud patches remain only a few days, others persist over a few months during the monsoon period[33]. The fluid mud patches are a seasonal phenomenon and do not appear at the same locations every year[31]; however, no large-scale migration pattern is observed.
Coast of the East China Sea

The East China Sea mud belt is located in deeper water than the two previous examples. It consists of fine clayey sediments originating mainly from the Yangtze River and transported to the south by the Zhejiang-Fujian Coastal Current (ZFCC). The fine sediments were deposited during the past 7000 years and form a bed layer of 10 to 40 m thick[34]. The deposit area is bounded at the seaward side by upwelling currents under the influence of various hydrodynamic processes. The dominant process, according to several studies, is the gravitational circulation driven by a sharp salinity gradient between the southward flowing Yangtze river plume and the northward flowing shelf currents (Taiwan Warm Current TWC and Kuroshio Current KC) [35][36]. During winter, sediments resuspended from the Yangtze River delta are carried to the mud belt by currents that also stir up sediments from the mud bed. However, suspended sediment concentrations are generally below 1 g/l and thus insufficient to form of a fluid mud layer capable to dampen incident waves[35]. Mud deposition therefore cannot occur on the shoreface of the East China coast; the mud belt is confined to the depth range between 20 and 50 m (Fig. 10).
Acknowledgements
The author is indebted to Han Winterwerp and Edward Anthony for numerous pertinent comments on an earlier version of this article.
Related articles
- Dynamics of mud transport
- Fluid mud
- Sediment deposition and erosion processes
- Chenier
- Characteristics of muddy coasts
- Estuarine turbidity maximum
- Coastal and marine sediments
- Ekman transport
References
- ↑ Porz, L., Zhang, W., Hanebuth, T.J.J. and Schrum, C. 2021. Physical processes controlling mud depocenter development on continental shelves – Geological, oceanographic, and modeling concepts. Marine Geology 432, 106402
- ↑ Winterwerp, J.C., de Graaff, R.F., Groeneweg, J. and Luijendijk, A.P. 2007. Modelling of wave damping at Guyana mud coast. Coastal Engineering 54: 249–261
- ↑ Elgar, S. and Raubenheimer, B. 2008. Wave dissipation by muddy seafloors. Geophysical Research Letters 35, L07611
- ↑ 4.0 4.1 4.2 4.3 Samiksha, S.V., Vethamony, P., Rogers, W.E., Pednekar, P.S., Babu, M.T and Dineshkumar, P.K. 2017. Wave energy dissipation due to mudbanks formed off southwest coast of India. Est. Coastal Shelf Science 196: 387-398 Cite error: Invalid
<ref>
tag; name "S17" defined multiple times with different content Cite error: Invalid<ref>
tag; name "S17" defined multiple times with different content Cite error: Invalid<ref>
tag; name "S17" defined multiple times with different content - ↑ 5.0 5.1 Eisma, D. 1966. Oceanographic observations on the Western Surinam shelf. Netherlands Institute for Sea Research, Rep. 1966-1
- ↑ Rao, D.S., Ramamirtham, C.P., Murty, A.V.S., Muthusamy, S., Kunhikrishnan N.P. and Khambadkar l.R. 1992. Oceanography of the Arabian sea with particular reference to the southwest monsoon. Bull. Cent. Mar. Fish. Res. Inst. 45: 4 – 8
- ↑ Hu, D. 1984. Upwelling and sedimentation dynamics. I. The role of upwelling in sedimentation in the Huanghai Sea and East China Sea—A description of general features. Chinese Journal of Oceanology and Limnology 2: 12-19
- ↑ Visser, M., de Ruijter, W.P.M. and Postma, L. 1991 The distribution of suspended matter in the Dutch coastal zone. Netherlands Journal of Sea Research 27: 127-143
- ↑ Souza, A.J. and Simpson, J.H. 1996. The modification of tidal ellipses by stratification in the Rhine ROFI. Continental Shelf Research 16: 997-1007
- ↑ van der Hout, C.M., Gerkema, T., Nauw, J.J. and Ridderinkhof, H. 2015. Observations of a narrow zone of high suspended particulate matter (SPM) concentrations along the Dutch coast. Continental Shelf Research 95: 27-38
- ↑ Flores, R.P., Rijnsburger, S., Horner-Devine, A.R., Kumar, N., Souza, A.J. and Pietrzak, J.D. 2020. The Formation of Turbidity Maximum Zones by Minor Axis Tidal Straining in Regions of Freshwater Influence. Journal Phys. Ocean. 50: 1265-1287
- ↑ Geyer, W.R., Hill, P.S. and Kineke, G.C. 2004. The transport, transformation and dispersal of sediment by buoyant coastal flows. Cont. Shelf Res. 24: 927–949
- ↑ Orseau, S., Zorrilla, N.A., Huybrechts, N., Lesourd, S., and Gardel, A. 2020. Decadal-scale morphological evolution of a muddy open coast. Marine Geology 420, 106048
- ↑ 14.0 14.1 14.2 Allison, M.A. and Lee, M.T. 2004. Sediment exchange between Amazon mudbanks and shore-fringing mangroves in French Guiana. Mar. Geol. 208: 169–190
- ↑ 15.0 15.1 15.2 15.3 Anthony, E. J., Gardel, A., Gratiot, N., Proisy, C., Allison, M. A., Dolique, F. and Formard, F. 2010. The Amazon-influenced muddy coast of South America: a review of mud bank-shoreline. Earth- Science Reviews 103: 99–121
- ↑ Augustinus, P.G.E.F. 2004.The influence of the trade winds on the coastal development of the Guianas at various scale levels: a synthesis. Mar.Geol. 208: 141–151
- ↑ Rao, D.S., Ramamirtham, C.P., Murty, A.V.S., Muthusamy, S., Kunhikrishnan N.P. and Khambadkar l.R. 1992. Oceanography of the arabian sea with particular reference to the southwest monsoon. Bull. Cent. Mar. Fish. Res. Inst. 45: 4 – 8
- ↑ 18.0 18.1 18.2 Parvathy, K., Noujas, V., Thomas, K. V. and Ramesh, H. 2015. Impact of Mudbanks on Coastal Dynamics. International Conference on Water Resources, Coastal and Ocean Engineering. Aquatic Procedia 4: 1514 – 1521
- ↑ Liu, J.T., Hsu, R.T., Yang, R.J., Wang, Y.P., Wu, H., Du, X., Li, A., Chien, S.C., Lee, J., Yang, S., Zhu, J., Su, C-C., Chang, Y. and Huh, C-A. 2018. A comprehensive sediment dynamics study of a major mud belt system on the inner shelf along an energetic coast. Scientific Reports 8:4229
- ↑ Wu, J., Ren, J., Liu, H., Qiu, C., Cui, Y. and Zhang, Q. 2015. Trapping and Escaping Processes of Yangtze River-derived Sediments to the East China Sea. Chapter in Geological Society London Special Publications
- ↑ Nizou, J., Hanebuth, T.J.J., Heslop, D., Schwenk, T., Palamenghi, L.,. Stuut, J-B. and Henrich, R. 2010. The Senegal River mud belt: A high-resolution archive of paleoclimatic change and coastal evolution. Marine Geology 278: 150–164
- ↑ Anthony, E.J. 2006 The muddy tropical coast of West Africa from Sierra Leone to Guinea-Bissau: geological heritage, geomorphology and sediment dynamics. Africa Geoscience Review 13: 227-237
- ↑ Bremner, J.M. 1980. Physical parameters of the diatomaceous mud belt off South West Africa. Marine Geology 34: M67--M76
- ↑ Kineke, G.C., Sternberg, R.W., Trowbridge, J.H. and Geyer, W.R., 1996. Fluid-mud processes on the Amazon continental shelf. Cont. Shelf Res. 16: 667–696
- ↑ Gratiot, N., Gardel, A. and Anthony, E.J. 2007. Trade-wind waves and mud dynamics on the French Guiana coast, South America: input from ERA-40 wave data and field investigations. Mar. Geol. 236: 15–26
- ↑ Eisma, D., Augustinus, P.G.E.F. and Alexander, C. 1991. Recent and subrecent changes in the dispersal of amazon mud. Neth. J. Sea Res. 28: 181–19
- ↑ Trowbridge J. H. and Kineke G. C. 1994. Structure and dynamics of fluid muds on the Amazon continental shelf. J. Geophys. Res. 99: 865-874
- ↑ Wells, J.T. and Kemp, G.P. 1986. Interaction of surface waves and cohesive sediments: field observations and geologic significance. In: Mehta, A.J. (Ed.), Estuarine Cohesive Sediment Dynamics, Lecture Notes on Coastal and Estuarine Studies. Springer New York, pp. 43–65
- ↑ Augustinus, P.G.E.F. 1980. Actual development of the chenier coast of Suriname (South America). Sedimentary Geology 26: 91—113
- ↑ Murray, A.B. and Thieler, E.R. 2004. A new hypothesis and exploratory model for the formation of large-scale inner-shelf sediment sorting and “rippled scour depressions”. Cont. Shelf Res. 24: 295–315
- ↑ 31.0 31.1 Philip, A.S., Babu, C.A. and Hareeshkumar, P.V. 2013. Meteorological aspects of mudbank formation along southwest coast of India. Continental Shelf Research 65: 45–51
- ↑ Shah, P., Sajeev, R. and Gopika, N. 2015. Study of Upwelling along the West Coast of India—A Climatological Approach. J. Coastal Res. 31: 1151–1158
- ↑ Rao, D.S., Ramamirtham, C.P., Murty, A.V.S., Muthusamy, S., Kunhikrishnan N.P. and Khambadkar l.R. 1992. Oceanography of the arabian sea with particular reference to the southwest monsoon. Bull. Cent. Mar. Fish. Res. Inst. 45: 4 – 8
- ↑ 34.0 34.1 Liu, J.P., Li, A.C., Xu, K.H., Velozzi, D.M., Yang, Z.S., Milliman, J.D. and DeMaster, D.J. 2006. Sedimentary features of the Yangtze River-derived along-shelf clinoform deposit in the East China Sea. Cont. Shelf Res. 26: 2141–2156
- ↑ 35.0 35.1 Li, Y.H., Qiao, L., Wang, A.J., Zhang, Y., Fang, J.Y. and Chen, J. 2013. Seasonal variation of water column structure and sediment transport in a mud depo-center off the Zhejiang-Fujian coast in China. Ocean Dyn. 63: 679–690
- ↑ Liu, Z., Gan, J., Wu, H., Hu, J., Cai, Z. and Deng, Y. 2021. Advances on Coastal and Estuarine Circulations Around the Changjiang Estuary in the Recent Decades (2000–2020). Front. Mar. Sci. 8, 615929
Please note that others may also have edited the contents of this article.
|